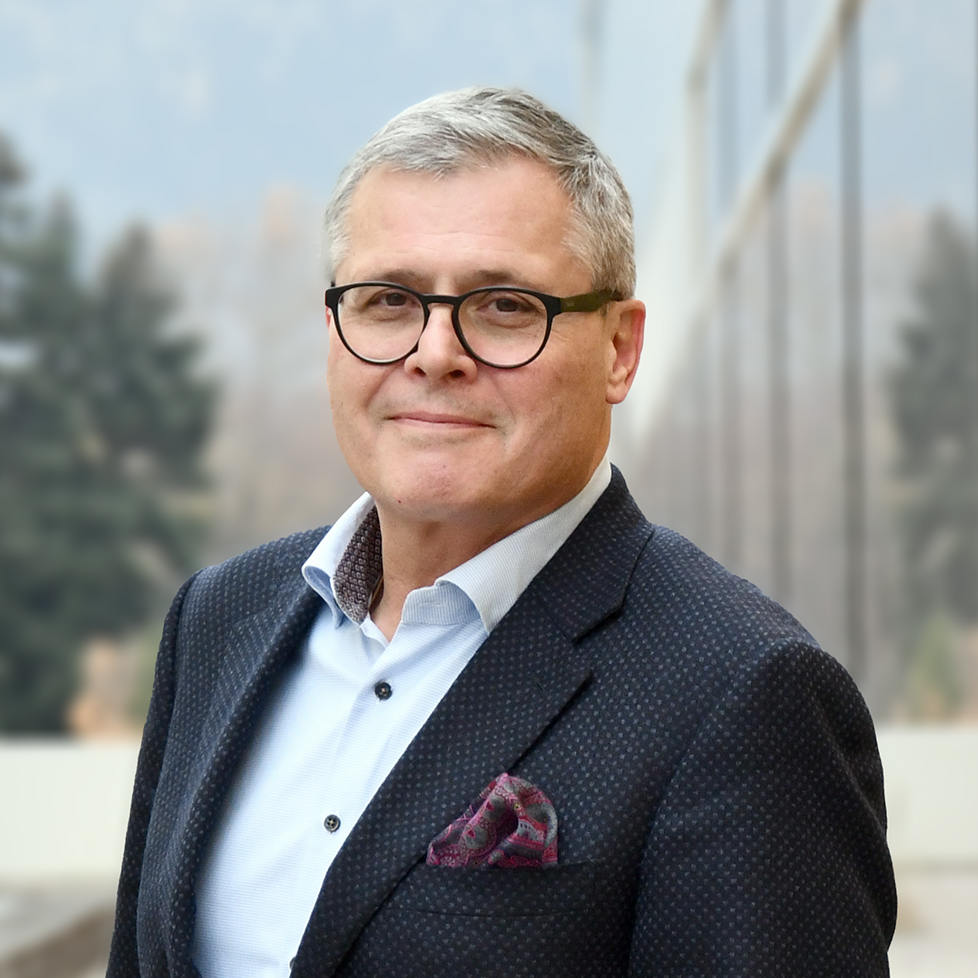
Innrain 80-82
6020 Innsbruck
Fax: +43 512 9003 74171
Email: Lukas.A.Huber@i-med.ac.at
Website: https://cellbiology.i-med.ac.at/
Research Branch (ÖSTAT Classification)
106013, 106023, 106037, 106041, 301904, 106052, 302035, 302016, 302025, 302078, 302084
Keywords
cancer, Cell biology, genetic model organisms, metabolic signalling, organelle proteostasis, protein transport and sorting, proteomics, and rare diseases
Research Focus
Rare diseases
Cancer
Membrane Proteostasis and Signalling
Cell Differentiation
Signal Transduction and Proteomics
General Facts
The institute studies molecular mechanisms that maintain cellular function and organisation. To address these fundamental questions, we use a combination of genetic model systems (yeast, mouse and human cells), microscopy and quantitative proteomics. We provide an international and dynamic research environment for Master and PhD students as well as postdoctoral fellows. We are an integral part of the international MCBD (Molecular and Cellular Biology of Disease) PhD programme and the Cellular Basis of Diseases (DOC82) doc.funds programme. In addition, we are involved in several EU projects and have numerous national and international collaborations with academic partners and biotech companies. We promote scientific partnership between basic research and hospitals.
Research
The Huber Laboratory: Lysosomal mTOR
Signalling – The Crossroads between Signal Transduction and Endosomal Biogenesis
Signal propagation in cells plays an essential role in the development of the tumour as well as in the course of the immune response and therefore in the development of metabolic disorders, such as diabetes and metabolic syndrome. LAMTOR, which was originally identified by our group, consists of seven proteins (p14, p18, MP1, HBXIP, p11 – also referred to as LAMTOR1-5 – a RagA and RagC). It coordinates cell division, cell growth, cell death, cell migration and lysosomal positioning (Filipek et al., J Cell Biol 2017) by recruiting the signalling proteins MAPK and mTORC to the lysosome. In a collaboration funded by the Austrian Science Fund (FWF), our research group, together with the group of Klaus Scheffzek and other colleagues at the Biocentre of the Medical University, have succeeded in elucidating the three-dimensional structure of the LAMTOR complex and its impact on signalling (Araujo et al., Science 2017). The LAMTOR1 subunit forms the clamp that binds the other components and tethers the protein complex to the lysosome, the mobile waste disposal and signalling platform of the cell. RagA and RagC, two signal components of the G protein family, are thus aligned with the mTORC signal path and therefore dock on to the lysosomal LAMTOR complex (Fig. 1).
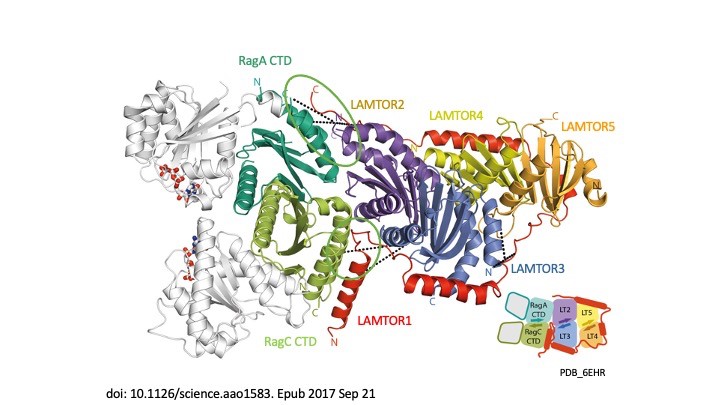
In collaboration with the group of Andrea Ballabio at TIGEM in Italy, we investigated the role of mTOR in a rare disease (Napolitano et al., Nature 2020). In 1977, three Canadian physicians discovered the eponymous rare autosomal-dominant Birt-Hogg-Dubé (BHD) syndrome. This disorder has been described in over 100 families throughout the world. The common feature here is the tendency to form kidney and lung cysts as well as skin lesions. The renal cysts often deteriorate and form malignant tumours. mTOR blocks the action of transcription factor TFEB during nutrient uptake. Conversely, TFEB is activated when there is a lack of nutrients and internal reserves have to be used. In this collaboration, we were able to identify for the first time that the renal phenotype (cysts and renal cell carcinoma) in BHD syndrome occurs as a result of constitutive activation of TFEB, which in turn leads to paradoxical mTORC1 hyper-activation in this disease.
The Teis Laboratory: Membrane Proteostasis and Signalling
The function of cells depends on the integrity of their membranes and organelles. To maintain membrane integrity selective protein degradation machineries detect and selectively degrade misfolded or orphaned proteins. By achieving this remarkable task, cellular quality-control networks maintain membrane protein homeostasis (proteostasis). Chronic defects membrane proteostasis causes loss of organelle integrity and cell injuries that are central to the pathophysiology of prevalent human diseases, including cancer, autoimmunity, diabetes, obesity and neurodegeneration. The function of selective protein degradation pathways is therefore a major issue in biology.
To tackle this issue, we aim to discover molecular mechanisms that connect cellular quality control processes to membrane lipid homeostasis in response to metabolic changes and cellular stress. To address these questions, my group combines genetic approaches in yeasts and human cells with quantitative biochemical and imaging approaches. Recently, we have (i) identified a novel membrane protein degradation pathway (EGAD) that selectively degrades membrane proteins in Golgi and endosomes to regulate sphingolipid homeostasis (Schmidt, O. EMBO J. 2019); (ii) characterised how metabolic signalling enlists adaptors for ubiquitin ligase complex to selectively degrade nutrient transporters (Ivashov V. et al., eLife 2020); (iii) discovered a role of the ESCRT machinery in regulating the interactions of chromosomes with the nuclear envelope (Pieper, G. et al Dev. Cell 2020) and (iv) described a function of the ESCRT machinery for maintaining the integrity of the plasma membrane (Schmidt, O. et al JBC. 2020).
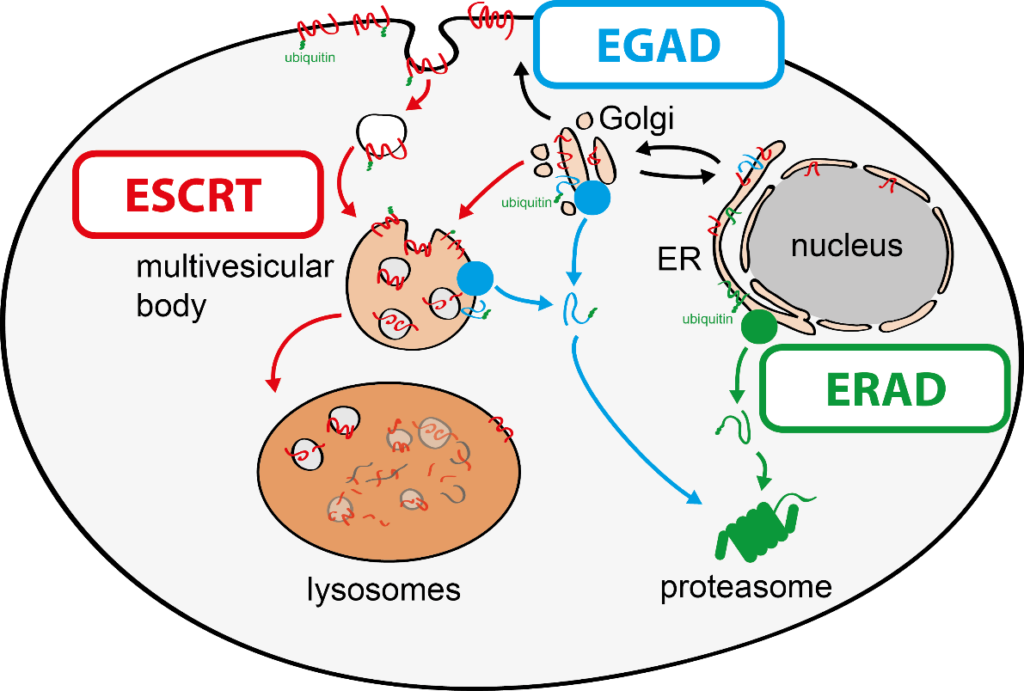
The Vogel Laboratory: Intracellular Cargo Transport in Congenital Intestinal Diseases
Our research focuses on congenital intestinal and liver diseases. Congenital intestinal disorders are often caused by mutations in genes that are essential for the correct intestinal-epithelial polarisation. One example is microvillus inclusion disease. Mutations in MYO5B or STX3 were identified here; both are essential for the polarised apical cargo transport of transmembrane transporters (Vogel GF et al., JCB, 2015; Vogel GF et al., JCI Insight, 2017). Now, there is also a new research focus on myo5b-associated cholestatic liver disease and myo5b-independent apical transport in intestinal epithelial cells.
Congenital liver disease can ultimately result in organ failure and require liver transplantation. However, the trigger and molecular mechanisms underlying liver failure remain scarcely understood. We are therefore studying the contribution of endoplasmic reticulum stress and the unfolded protein response in metabolic liver diseases and associated acute liver failure. Mutations in PERK are a prominent example here, associated with Wolcott-Rallison syndrome. Liver and intestinal diseases are both studied on genome-edited epithelial cell lines as well as patient-derived organoid cultures.
In close collaboration with Andreas Janecke and Thomas Müller from Paediatrics I, we recently identified two novel missense mutations in the adaptor protein AP1S1 in patients diagnosed with congenital enteropathy. Defects in AP1S1 are associated with MEDNIK syndrome. In AP1S1 knockout CaCo2 cells, we observed altered localisation of tight-junction proteins ZO-1 and Claudin 3, reduced trans-epithelial electrical resistance and increased dextran permeability of the CaCo2-AP1S1-KO monolayer. In addition, lumen formation in 3D cultures of these cells was abnormal. Re-expression of wild-type AP1S1 in CaCo2-AP1S1-KO cells reversed these abnormalities, not so the expression of AP1S1 that contained either missense mutation. Our data indicate that loss of AP1S1 function causes an intestinal epithelial barrier defect.
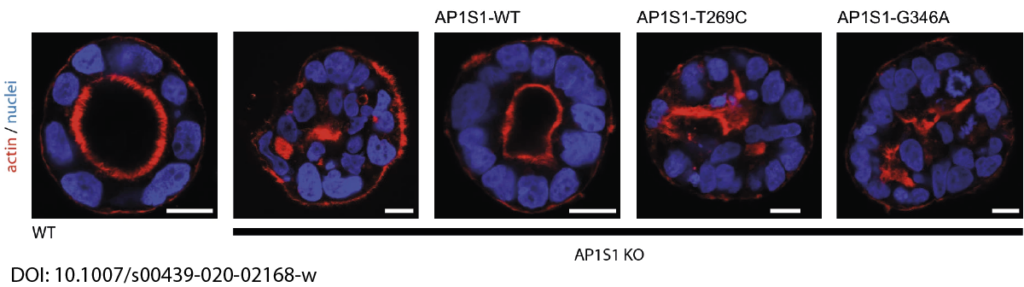
The Vietor Laboratory: Regulatory Mechanisms of Cell Differentiation
TPA-induced sequence-7 (TIS7) protein has been shown to be involved in the regulation of differentiation processes in various cell types, e.g. neurons, enterocytes, myocytes and recently also adipocytes. Current data show that TIS7 is upregulated in white adipose tissues of genetically obese ob/ob mice. In previous studies, we have identified that TIS7 protein functions as a transcriptional co-regulator, owing to its interaction with protein complexes that contain either histone deacetylases or protein methyl transferases (Lammirato et al., BMC Biol, 2016). Analyses of adipocyte differentiation suggested an involvement of TIS7 in the regulation of adipogenesis in the Wnt/β-catenin signalling context. Based on experiments with TIS7 knockout mice generated in our laboratory, we identified a negative effect of TIS7 on Wnt signalling and a positive effect on adipocyte differentiation. Compared with wild-type littermates, TIS7 knockout mice do not gain weight when chronically fed with a high-fat diet. SKMc15, a second member of TIS7 gene family, is highly conserved in different species. However, there has been no information concerning the physiological function and mechanisms of action of SKMc15 and its possible involvement in the differentiation of various tissues to date. We have therefore utilised mice that lack either SKMc15 or both TIS7 and SKMc15 genes and are currently analysing the effects of both TIS7 and SKMc15 on the regulation of adipogenesis. The ablation of both TIS7 and SKMc15 genes significantly affected the expression of genes that are essential for adipocyte differentiation and function. Since dKO mice render a substantially leaner phenotype, we propose that TIS7 and SKMc15 represent novel players in the process of physiological adipocyte differentiation.
Differentiated cells can re-enter the cell cycle to repair tissue damage, via a series of discrete morphological and molecular stages. Using TIS7 knockout mice generated in our laboratory as a specific experimental animal model, the group with which we are involved, led by Prof. Jason C Mills from Washington University School of Medicine in St Louis, USA, identified TIS7 as a part of this conserved cellular regeneration programme called “paligenosis”.
We were recently involved in the development of a novel assay in Taras Valovka’s laboratory, to detect and characterise interactions between transcription factor NF-κB and dsDNA. This technique can be expanded to detect and characterise sequence-dependent protein-dsDNA interactions as well as other transcriptional factors. Together with the Ezzatollah Fathi laboratory at the University of Tabriz, Iran, we studied various cellular mechanisms involved in tissue regeneration, with a possible application in experimental cell therapies. This project resulted in a number of joint publications in 2020.
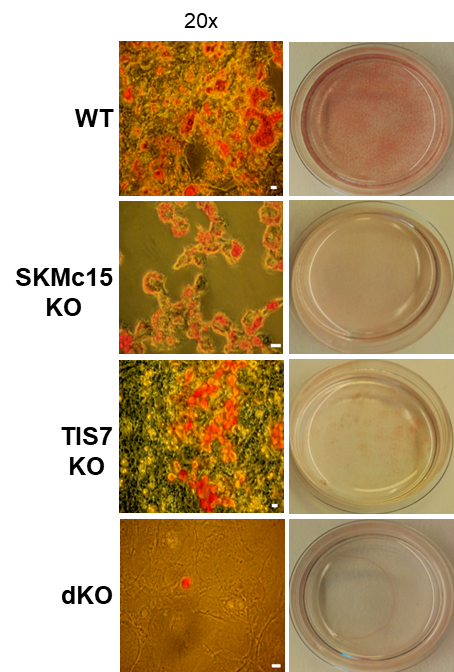
The Schmidt laboratory: Regulation of cellular lipid homeostasis
Sphingolipids are an essential class of lipids and structural components of all cellular membranes. They are indispensable for myelination in the nervous system, and they are also important signalling molecules. The biosynthesis of sphingolipids is initiated at the endoplasmic reticulum (ER) with the condensation of L-serine and palmitic acid by the enzyme serine-palmitoyl-coenzyme A transferase (SPT). This reaction is rate-limiting for sphingolipid synthesis and tightly regulated, as the accumulation of sphingolipids or their synthesis intermediates in particular is detrimental for cells and associated with a number of diseases frequently including neurodegeneration (e.g. Gaucher and Fabry’s disease or hereditary sensory and autonomic neuropathy type 1 (HSAN1)).
SPT activity is regulated by the evolutionary conserved family of Orm1-like proteins, of which humans possess three paralogues (ORMDL1-3). They are membrane proteins of the ER associating with the SPT and thereby inhibit its catalytic activity. Why the genomes of most organisms encode more for than one ORMDL paralogue is not clear. Genetic mutations affecting the interaction of ORMDL family proteins with the SPT are of high pathological relevance. The loss of this regulatory module and hence uncontrolled synthesis of sphingolipid precursors may be causative for a subtype of amyotrophic lateral sclerosis (ALS), whereas increased expression of ORMDL3 is associated with several common inflammatory diseases, including childhood asthma and IBD.
The regulation of SPT by ORMDL proteins is best characterised in the budding yeast S. cerevisiae. Yeast has two ORMDL proteins, Orm1 and Orm2, which are under the control of plasma membrane stress signalling via the protein kinases TORC2 and Ypk1. Ypk1 phosphorylation causes dissociation of Orm1/2 and activates the SPT complex; however, this phospho-regulation is only conserved in fungal species. Recently, we described an additional level of regulation of Orm proteins by translocation from the ER and degradation by the endosome and Golgi-associated degradation (EGAD; Schmidt et al., 2019, EMBO J.; Schmidt et al., 2020, JBC). This is the first reported example of a mode of regulation that is not shared by all ORMDL paralogs, as only Orm2 is exported and degraded while Orm1 remains stable. Of note, human ORMDL protein levels are also regulated by proteolysis (Sasset et al., 2023).
In the Schmidt lab, we study the regulation of SPT by ORMDL-family proteins particularly in light of differences between the paralogs, to understand why life typically relies on more than one ORMDL protein. We attempt to address fundamental research questions initially in budding yeast as an easily controllable model system and subsequently see whether they are conserved in humans. It is our goal to discover how individual ORMDL paralogues may contribute to the homeostatic regulation of sphingolipid metabolism and how their malfunction leads to human diseases.
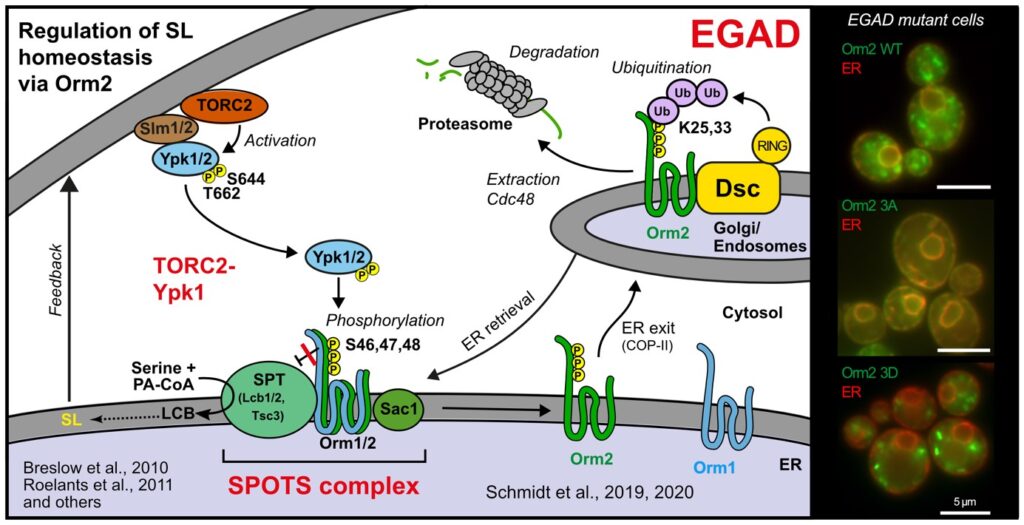
Golgi-associated degradation; 3A, non-phosphorylatable Orm2 mutant; 3D, phospho-mimetic Orm2 mutant)
The Valovka Laboratory: Molecular Basis of Rare Genetic Disorders
Our research deals with the clarification of molecular mechanisms implicated in rare, inherited diseases. Rare diseases are usually caused by genetic mutations in a broad spectrum of genes, ranging from metabolic enzymes to those that regulate the immune function or those that cause cancer, which can affect almost any organ system in humans. As a part of the cooperation with the Department of Paediatrics I at MUI (Andreas Janecke and Thomas Müller) in recent years, we have identified and studied several novel pathogenic mutations in various genes, including PCNT, ACTB, DOCK7, RIPK1, and UNC45A (Waich et al., Clinical Genetics 2020; Baumann et al., Hum Mutat 2020; Haberlandt et al., Mol Genet Genomic Med 2021; Duclaux-Loras et al., J Clin Invest 2022).
Novel PCNT Variants with an Unusual Clinical Presentation of MOPDII
Microcephalic osteodysplastic primordial dwarfism type II (MOPDII) is a rare autosomal recessive disorder characterised by extreme pre and postnatal growth restriction, skeletal dysplasia, dental anomalies and craniofacial dysmorphism, and often mild mental retardation. Biallelic loss‐of‐function mutations in the pericentrin gene (PCNT) have been defined as the cause of the disease. Here, we described a new case of MOPDII with an unusual, attenuated growth restriction and pachygyria. Compound heterozygosity for novel truncated PCNT variants was identified in a family with two affected siblings. Our studies revealed the expression of both mutated PCNT variants and showed their ability to partially retain the centrosome-associated functions in patient fibroblasts (Fig. 6). These findings explain the mild manifestation of MOPDII described and expand our knowledge of the clinical and molecular spectrum of this disease.
Novel Methodological Approach to Inhibitory Screening
Screening for synthetic and natural anti-inflammatory compounds forms another aspect of our research. In collaboration with the Austrian Drug Screening Institute (ADSI), we established a screening platform to identify and isolate anti-inflammatory bioactive compounds in natural extracts. By using this platform, we have identified and thoroughly characterised a number of algae strains from the ASIB 505 terrestrial algae collection (the Institute of Botany, LFUI; the MCI – Management Center Innsbruck) that had unique anti-inflammatory properties (Leitner et al., Cells 2022; EU Patent application: EP4104845). In the context of this work, we also developed a high-throughput, fluorescent thermal shift-based method of detecting nuclear factor kappa B (NF-κB) binding to double stranded DNA (Leitner et al., Sci Rep 2021). This method allows the measurement of dose-dependent inhibitory effects on NF-κB DNA binding as well as the accurate calculation of equilibrium binding constants.
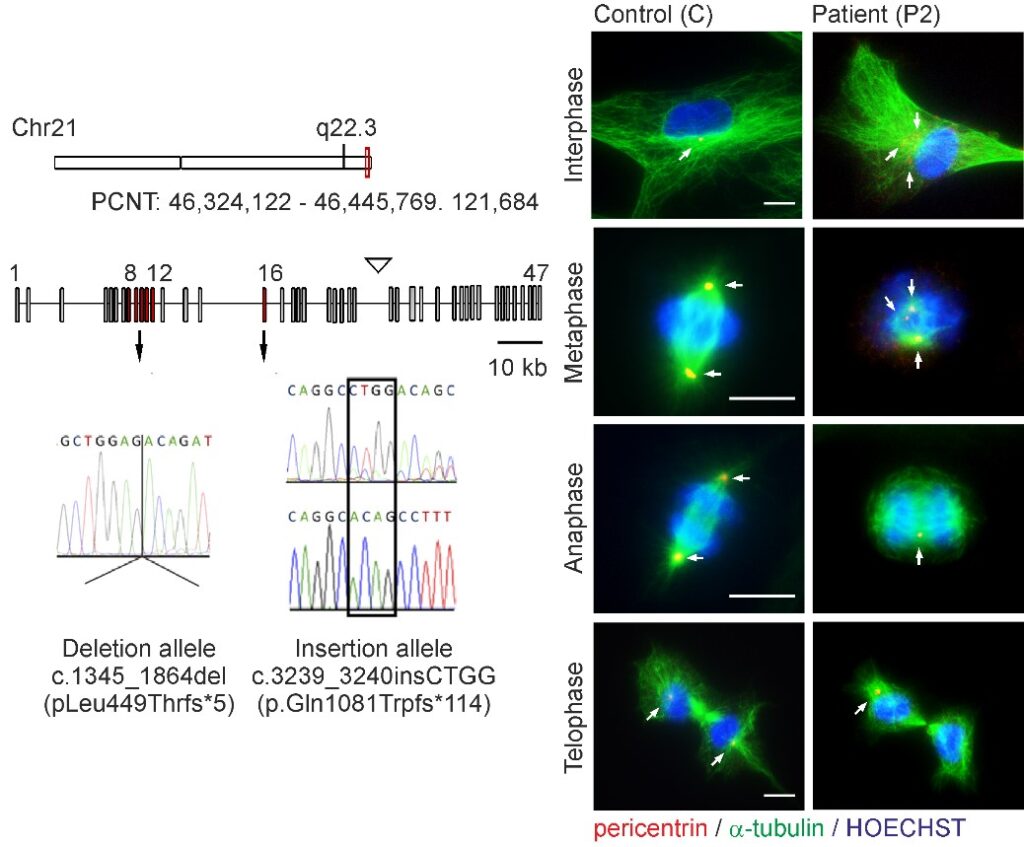
The Araujo Laboratory: Lysosomal signalling complexes – Coordinating organelle function with cellular homeostasis
Lysosomes are membrane-bound organelles that fulfil several functions, from protein degradation to the control of cellular metabolism. Due to their importance for overall proteostasis and signalling, cells have developed unique mechanisms to control lysosomal numbers, size, movement, degradative capacity, interaction with other organelles and even Iysophagy, the name given to the selective degradation of dysfunctional lysosomes. The importance of this organelle is highlighted by the variety of diseases known to be associated with lysosomal abnormalities, from monogenic lysosomal storage disorders to brain pathologies, metabolic disorders, cancer and aging.
One of the key signalling complexes present in the lysosomal membrane is mTORC1, the mechanistic target of rapamycin complex 1. It regulates the balance between biosynthetic and catabolic processes influencing a plethora of cellular processes. Due to the fact that mTORC1 activation triggers a fairly resource-intensive anabolic program, cells have evolved mechanisms to ensure that it only becomes active when sufficient resources are available. Over the last years, works from a number of laboratories have elucidated many aspects of mTORC1’s regulation: from identifying the key players to deciphering the molecular mechanisms involved and the three-dimensional structure of these assemblies.
Building on this vast pool of knowledge, we focus on the interplay between canonical mTORC1 signalling components and other lysosomal proteins/complexes. Our objectives are three fold:
1) Decipher how these molecular machineries coordinate their activity and help control the organelle’s properties and function.
2) Determine the implication of these events for overall cellular homeostasis.
3) Understand how dysfunction of these processes is the root of different disease conditions.
Overall, we strongly believe that scientists will eventually be able to offer reasonable therapeutic options for patients with rare disease mutations in lysosomal genes only through a deeper understanding of the interplay between these lysosomal machineries.
To address this issue, we combine genome editing with cell biology techniques, biochemical and imaging approaches. We also collaborate actively with structural biology groups, to shed light on specific molecular mechanisms controlling our favourite proteins.
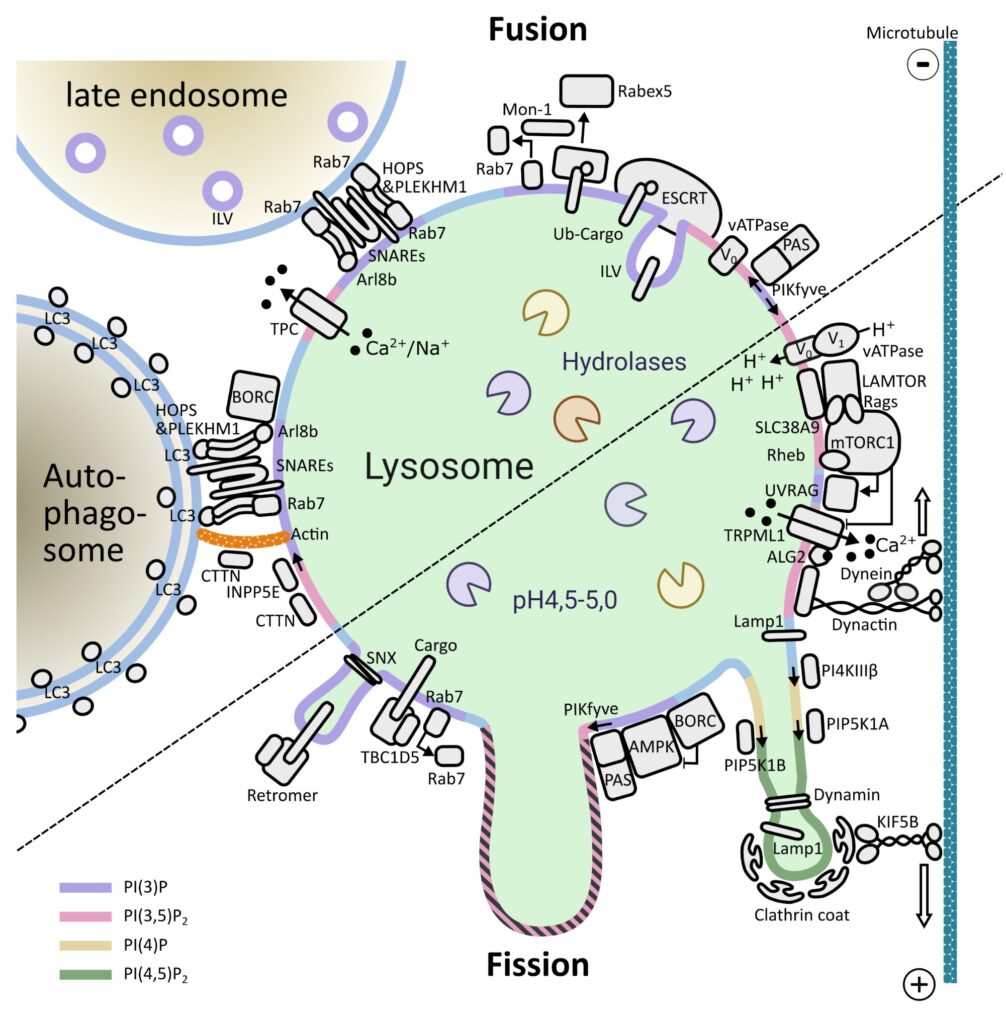
Pictures
Selected Publications
The Huber Laboratory: Lysosomal mTOR Signalling – The Crossroads between Signal Transduction and Endosomal Biogenesis
Structure of the lysosomal mTORC1-TFEB-Rag-Ragulator megacomplex. Cui Z, Napolitano G, de Araujo MEG, Esposito A, Monfregola J, Huber LA, Ballabio A, Hurley JH.Nature. 2023 Jan 25. doi: 10.1038/s41586-022-05652-7. Online ahead of print. PMID: 36697823
A substrate-specific mTORC1 pathway underlies Birt-Hogg-Dubé syndrome. Napolitano G, Di Malta C, Esposito A, de Araujo MEG, Pece S, Bertalot G, Matarese M, Benedetti V, Zampelli A, Stasyk T, Siciliano D, Venuta A, Cesana M, Vilardo C, Nusco E, Monfregola J, Calcagnì A, Di Fiore PP, Huber LA, Ballabio A. Nature. 2020 Sep;585(7826):597-602. doi: 10.1038/s41586-020-2444-0. Epub 2020 Jul 1. PMID: 32612235
LAMTOR/Ragulator is a negative regulator of Arl8b- and BORC-dependent late endosomal positioning. Filipek PA, de Araujo MEG, Vogel GF, De Smet CH, Eberharter D, Rebsamen M, Rudashevskaya EL, Kremser L, Yordanov T, Tschaikner P, Fürnrohr BG, Lechner S, Dunzendorfer-Matt T, Scheffzek K, Bennett KL, Superti-Furga G, Lindner HH, Stasyk T, Huber LA. J Cell Biol. 2017 Dec 4;216(12):4199-4215. doi: 10.1083/jcb.201703061. Epub 2017 Oct 9. PMID: 28993467 Free PMC article.
Crystal structure of the human lysosomal mTORC1 scaffold complex and its impact on signaling. de Araujo MEG, Naschberger A, Fürnrohr BG, Stasyk T, Dunzendorfer-Matt T, Lechner S, Welti S, Kremser L, Shivalingaiah G, Offterdinger M, Lindner HH, Huber LA, Scheffzek K. Science. 2017 Oct 20;358(6361):377-381. doi: 10.1126/science.aao1583. Epub 2017 Sep 21. PMID: 28935770
The Teis Laboratory: Membrane Proteostasis and Signalling
Complementary α-arrestin-ubiquitin ligase complexes control nutrient transporter endocytosis in response to amino acids. Ivashov V, Zimmer J, Schwabl S, Kahlhofer J, Weys S, Gstir R, Jakschitz T, Kremser L, Bonn GK, Lindner H, Huber LA, Leon S, Schmidt O, Teis D. Elife. 2020 Aug 3;9:e58246. PMID:32744498
ESCRT-III/Vps4 Controls Heterochromatin-Nuclear Envelope Attachments. Pieper GH, Sprenger S, Teis D, Oliferenko S. Dev Cell. 2020 Apr 6;53(1):27-41.e6.. PMID:32109380
Endosome and Golgi-associated degradation (EGAD) of membrane proteins regulates sphingolipid metabolism. Schmidt O, Weyer Y, Baumann V, Widerin MA, Eising S, Angelova M, Schleiffer A, Kremser L, Lindner H, Peter M, Fröhlich F, Teis D. EMBO J. 2019 Aug 1;38(15):e101433. PMID:31368600
TOR complex 2 (TORC2) signalling and the ESCRT machinery cooperate in the protection of plasma membrane integrity in yeast. Schmidt O, Weyer Y, Sprenger S, Widerin MA, Eising S, Baumann V, Angelova M, Loewith R, Stefan CJ, Hess MW, Fröhlich F, Teis D.J Biol Chem. 2020 Aug 21;295(34):12028-12044. PMID: 32611771
The Vogel Laboratory: Intracellular Cargo Transport in Congenital Intestinal Diseases
A CRISPR-screen in intestinal epithelial cells identifies novel factors for polarity and apical transport.
Klee KMC, Hess MW, Lohmüller M, Herzog S, Pfaller K, Müller T, Vogel GF*, Huber LA. Elife. 2023 Jan 20;12:e80135. doi: 10.7554/eLife.80135. Online ahead of print. PMID: 36661306
*Corresponding author
AP1S1 missense mutations cause a congenital enteropathy via an epithelial barrier defect. Klee KMC, Janecke AR, Civan HA, Rosipal Š, Heinz-Erian P, Huber LA, Müller T, Vogel GF.Hum Genet. 2020 Oct;139(10):1247-1259. doi: 10.1007/s00439-020-02168-w. Epub 2020 Apr 18.PMID: 32306098Free PMC article.
Co-existence of ABCB11 and DCDC2 disease: Infantile cholestasis requires both next-generation sequencing and clinical-histopathologic correlation. Vogel GF, Maurer E, Entenmann A, Straub S, Knisely AS, Janecke AR, Müller T.Eur J Hum Genet. 2020 Jun;28(6):840-844. doi: 10.1038/s41431-020-0613-0. Epub 2020 Mar 20.PMID: 32203204
The haemochromatosis gene Hfe and Kupffer cells control LDL cholesterol homeostasis and impact on atherosclerosis development. Demetz E, Tymoszuk P, Hilbe R, Volani C, Haschka D, Heim C, Auer K, Lener D, Zeiger LB, Pfeifhofer-Obermair C, Boehm A, Obermair GJ, Ablinger C, Coassin S, Lamina C, Kager J, Petzer V, Asshoff M, Schroll A, Nairz M, Dichtl S, Seifert M, von Raffay L, Fischer C, Barros-Pinkelnig M, Brigo N, Valente de Souza L, Sopper S, Hirsch J, Graber M, Gollmann-Tepeköylü C, Holfeld J, Halper J, Macheiner S, Gostner J, Vogel GF, Pechlaner R, Moser P, Imboden M, Marques-Vidal P, Probst-Hensch NM, Meiselbach H, Strauch K, Peters A, Paulweber B, Willeit J, Kiechl S, Kronenberg F, Theurl I, Tancevski I, Weiss G.Eur Heart J. 2020 Oct 21;41(40):3949-3959. doi: 10.1093/eurheartj/ehaa140.PMID: 32227235
The Vietor Laboratory: Regulatory Mechanisms of Cell Differentiation
A Dedicated Evolutionarily Conserved Molecular Network Licenses Differentiated Cells to Return to the Cell Cycle. Miao ZF, Lewis MA, Cho CJ, Adkins-Threats M, Park D, Brown JW, Sun JX, Burclaff JR, Kennedy S, Lu J, Mahar M, Vietor I, Huber LA, Davidson NO, Cavalli V, Rubin DC, Wang ZN, Mills JC.Dev Cell. 2020 Oct 26;55(2):178-194.e7. doi: 10.1016/j.devcel.2020.07.005. Epub 2020 Aug 7.PMID: 32768422
LAMTOR/Ragulator regulates lipid metabolism in macrophages and foam cell differentiation. Lamberti G, De Smet CH, Angelova M, Kremser L, Taub N, Herrmann C, Hess MW, Rainer J, Tancevski I, Schweigreiter R, Kofler R, Schmiedinger T, Vietor I, Trajanoski Z, Ejsing CS, Lindner HH, Huber LA, Stasyk T.FEBS Lett. 2020 Jan;594(1):31-42. doi: 10.1002/1873-3468.13579. Epub 2019 Aug 26.PMID: 31423582
Fluorescent thermal shift‑based method for detection of NF‑κB binding to double‑stranded DNA. Leitner PD, Vietor I, Huber LA, Valovka T. Sci Rep. 2021 Jan 27;11(1):2331. doi: 10.1038/s41598-021-81743-1.PMID: 33504856
The Schmidt laboratory: Regulation of cellular lipid homeostasis
TOR complex 2 (TORC2) signaling and the ESCRT machinery cooperate in the protection of plasma membrane integrity in yeast. Schmidt O*, Weyer Y, Sprenger S, Widerin MA, Eising S, Baumann V, Angelova M, Loewith R, Stefan CJ, Hess MW, Fröhlich F, Teis D. J Biol Chem. 2020 Aug 21;295(34):12028-12044. doi: 10.1074/jbc.RA120.013222. Epub 2020 Jul 1. PMID: 32611771
Endosome and Golgi-associated degradation (EGAD) of membrane proteins regulates sphingolipid metabolism. Schmidt O, Weyer Y, Baumann V, Widerin MA, Eising S, Angelova M, Schleiffer A, Kremser L, Lindner H, Peter M, Fröhlich F, Teis D. EMBO J. 2019 Aug 1;38(15):e101433. doi: 10.15252/embj.2018101433. Epub 2019 May 27. PMID:31368600
Complementary α-arrestin-ubiquitin ligase complexes control nutrient transporter endocytosis in response to amino acids. Ivashov V, Zimmer J, Schwabl S, Kahlhofer J, Weys S, Gstir R, Jakschitz T, Kremser L, Bonn GK, Lindner H, Huber LA, Leon S, Schmidt O*, Teis D. 2020 Aug 3;9:e58246. doi: 10.7554/eLife.58246.PMID: 32744498
The Valovka Laboratory: Molecular Basis of Rare Genetic Disorders
Novel PCNT variants in MOPDII with attenuated growth restriction and pachygyria. Waich S, Janecke AR, Parson W, Greber-Platzer S, Müller T, Huber LA, Valovka T, Vodopiutz J. Clin Genet. 2020 Sep;98(3):282-287. doi: 10.1111/cge.13797. Epub 2020 Jul 7. PMID: 32557621
Characteristic facial features and cortical blindness distinguish the DOCK7-related epileptic encephalopathy. Haberlandt E, Valovka T, Janjic T, Müller T, Blatsios G, Karall D, Janecke AR. Mol Genet Genomic Med. 2021 Jan 20:e1607. doi: 10.1002/mgg3.1607. Online ahead of print. PMID: 33471954
Fluorescent thermal shift-based method for detection of NF-κB binding to double-stranded DNA. Leitner PD, Vietor I, Huber LA, Valovka T. Sci Rep. 2021 Jan 27;11(1):2331. doi: 10.1038/s41598-021-81743-1.PMID: 33504856
Anti-Inflammatory Extract from Soil Algae Chromochloris zofingiensis Targeting TNFR/NF-κB Signaling at Different Levels. Leitner PD, Jakschitz T, Gstir R, Stuppner S, Perkams S, Kruus M, Trockenbacher A, Griesbeck C, Bonn GK, Huber LA, Valovka T. Cells. 2022 Apr 21;11(9):1407. doi: 10.3390/cells11091407. PMID: 35563717
UNC45A deficiency causes microvillus inclusion disease-like phenotype by impairing myosin VB-dependent apical trafficking. Duclaux-Loras R, Lebreton C, Berthelet J, Charbit-Henrion F, Nicolle O, Revenu de Courtils C, Waich S, Valovka T, Khiat A, Rabant M, Racine C, Guerrera IC, Baptista J, Mahe MM, Hess MW, Durel B, Lefort N, Banal C, Parisot M, Talbotec C, Lacaille F, Ecochard-Dugelay E, Demir AM, Vogel GF, Faivre L, Rodrigues A, Fowler D, Janecke AR, Müller T, Huber LA, Rodrigues-Lima F, Ruemmele FM, Uhlig HH, Del Bene F, Michaux G, Cerf-Bensussan N, Parlato M.J Clin Invest. 2022 May 16;132(10):e154997. doi: 10.1172/JCI154997.PMID: 35575086
The Araujo Laboratory: Lysosomal signaling complexes – Coordinating organelle function with cellular homeostasis
Structure of the lysosomal mTORC1-TFEB-Rag-Ragulator mega complex. Cui Z, Napolitano G, de Araujo MEG, Esposito A., Monfregola J, Huber LA, Ballabio A and Hurley JH. Nature 2023, Feb;614(7948):572-579. doi: 10.1038/s41586-022-05652-7.
Establishing spatial control over TORC1 signaling.Schmidt O, de Araujo MEG. J Cell Biol, 2022 May 2;221(5):e202203136.doi: 10.1083/jcb.202203136.
The SZT2 Interactome Unravels New Functions of the KICSTOR Complex. Cattelani C, Lesiak D, Liebscher G, Singer I, Stasyk T, Wallnöfer MH, Heberle AM, Corti C, Hess MW, Pfaller K, Kwiatkowski M, Pramstaller PP, Hicks AA, Thedieck K, Müller T, Huber LA and de Araujo MEG. Cells 2021, 10(10), 2711; https://doi.org/10.3390/cells10102711
Overcoming limitations in the availability of swabs systems used for SARS-CoV-2 laboratory diagnostics. Nairz M, Bellmann-Weiler R, Ladstätter M, Schüllner F, Zimmermann M, Koller AM, Blunder S, Naschberger H, Klotz W, Herold M, Kerndler S, Jeske M, Haschka D, Petzer V, Schroll A, Sonnweber T, Tancevski I, Fritsche G, de Araujo MEG, Stasyk T, Huber LA, Griesmacher A, Theurl I, Weiss G. Sci Rep. 2021 Jan 26;11(1):2261. doi: 10.1038/s41598-021-81782-8.PMID: 33500503
G3BPs tether the TSC complex to lysosomes and suppress mTORC1 signaling. Prentzell MT, Rehbein U, Cadena Sandoval M, De Meulemeester AS, Baumeister R, Brohée L, Berdel B, Bockwoldt M, Carroll B, Chowdhury SR, von Deimling A, Demetriades C, Figlia G; Genomics England Research Consortium, de Araujo MEG, Heberle AM, Heiland I, Holzwarth B, Huber LA, Jaworski J, Kedra M, Kern K, Kopach A, Korolchuk VI, van ‘t Land-Kuper I, Macias M, Nellist M, Palm W, Pusch S, Ramos Pittol JM, Reil M, Reintjes A, Reuter F, Sampson JR, Scheldeman C, Siekierska A, Stefan E, Teleman AA, Thomas LE, Torres-Quesada O, Trump S, West HD, de Witte P, Woltering S, Yordanov TE, Zmorzynska J, Opitz CA, Thedieck K Cell. 2021 Jan 18:S0092-8674(20)31694-9. doi: 10.1016/j.cell.2020.12.024. PMID: 33497611
Selection of Funding
The Huber Laboratory: Lysosomal mTOR Signalling – The Crossroads Between Signal Transduction and Endosomal Biogenesis
FWF P 32608 and P 26682, and FWF DOC 82 doc.fund Cellular Basis of Diseases: Molecular Control of Metabolism and Inflammation
The Teis Laboratory: Membrane Proteostasis and Signalling
FWF P 32161, P 30263 and P29583
Coordination of FWF Doctoral Program DOC82: Cellular Basis of Diseases: Molecular Control of Metabolism and Inflammation
The Vogel Laboratory: Intracellular Cargo Transport in Congenital Intestinal Diseases
ÖNB Anniversary Fund 18019, TWF Grant 0404/2386, ÖGKJ research grant
The Vietor Laboratory: Regulatory Mechanisms of Cell Differentiation
IMPULSE Iran Austria
The Schmidt laboratory: Regulation of cellular lipid homeostasis
Lipotype – Lipid excellence award 2019 (#2)
FWF P36187-B “individual functions of Orm/ORMDL family proteins in membrane homeostasis”
The Araujo Laboratory: Lysosomal signalling complexes – Coordinating organelle function with cellular homeostasis
FWF FG 2005/61357 – 2023- Organelle proteostasis in cellular quiescence and growth
TWF GZ F16676/5-2019 – A potential new mechanism for selective modulation of mTORC1
Collaborations
The Huber Laboratory: Lysosomal mTOR Signalling – The Crossroads between Signal Transduction and Endosomal Biogenesis
Giulio Suerti-Furga CeMM, David Haselbach IMP, Manuela Baccarini Max Perutz Laboratories all Vienna Austria
Dagmar Kratky Gottfried Schatz Research Center, Molecular Biology and Biochemistry, Medical University of Graz, AUT
Andrea Ballabio TIGEM, Naples, Italy
Structural Membrane Biology, Jinm Hurley University of California, Berkely, US
The Teis Laboratory: Membrane Proteostasis and Signalling
Robbie Loewith, University of Geneva, Geneva, CH
Snezhana Oliferenko, Francis Crick Institute, King’s College, UK
Benoit Kornmann, University Oxford, Oxford, UK
Tomas Kirchhausen, Harvard Medical School, Cambridge, USA
Scott Emr, Cornell University, Ithaca, UK
Florian Fröhlich, University Osnabrück, Osnabrück, DE
Sebastian Leon, Université de Paris, Paris, F
The Vogel Laboratory: Intracellular Cargo Transport in Congenital Intestinal Diseases
Saskia Wortmann, Paediatrics, University Hospital Salzburg, AT
The Vietor Laboratory: Regulatory Mechanisms of Cell Differentiation
Ezzatollah Fathi, Department of Clinical Sciences, Faculty of Veterinary Medicine, University of Tabriz, Tabriz, Iran
The Schmidt laboratory: Regulation of cellular lipid homeostasis
Florian Fröhlich, Osnabrück University, DE
Maria Bohnert, Münster University, DE
Robbie Loewith, University of Geneva, CH
Matthias Peter, ETH Zurich, CH
The Valovka Lab: Molecular Basis of Rare Genetic Disorders
The Austrian Drug Screening Institute (ADSI), AUT
Christoph Griesbeck, Department of Biotechnology and Food Engineering, MCI – The Entrepreneurial School, AUT
Julia Vodopiutz, Department of Pediatrics and Adolescent Medicine, Medical University of Vienna, AUT
Daniel Kotlarz, Department of Pediatrics, Ludwig-Maximilians-Universität (LMU) Munich, DE
The Araujo Laboratory: Lysosomal signaling complexes – Coordinating organelle function with cellular homeostasis
Gennaro Napolitano (Tigem, Naples, IT)
David Haselbach (IMP, Vienna, AUT)
Kathrin Thedieck (Institute of Biochemistry,University of Innsbruck, AUT)