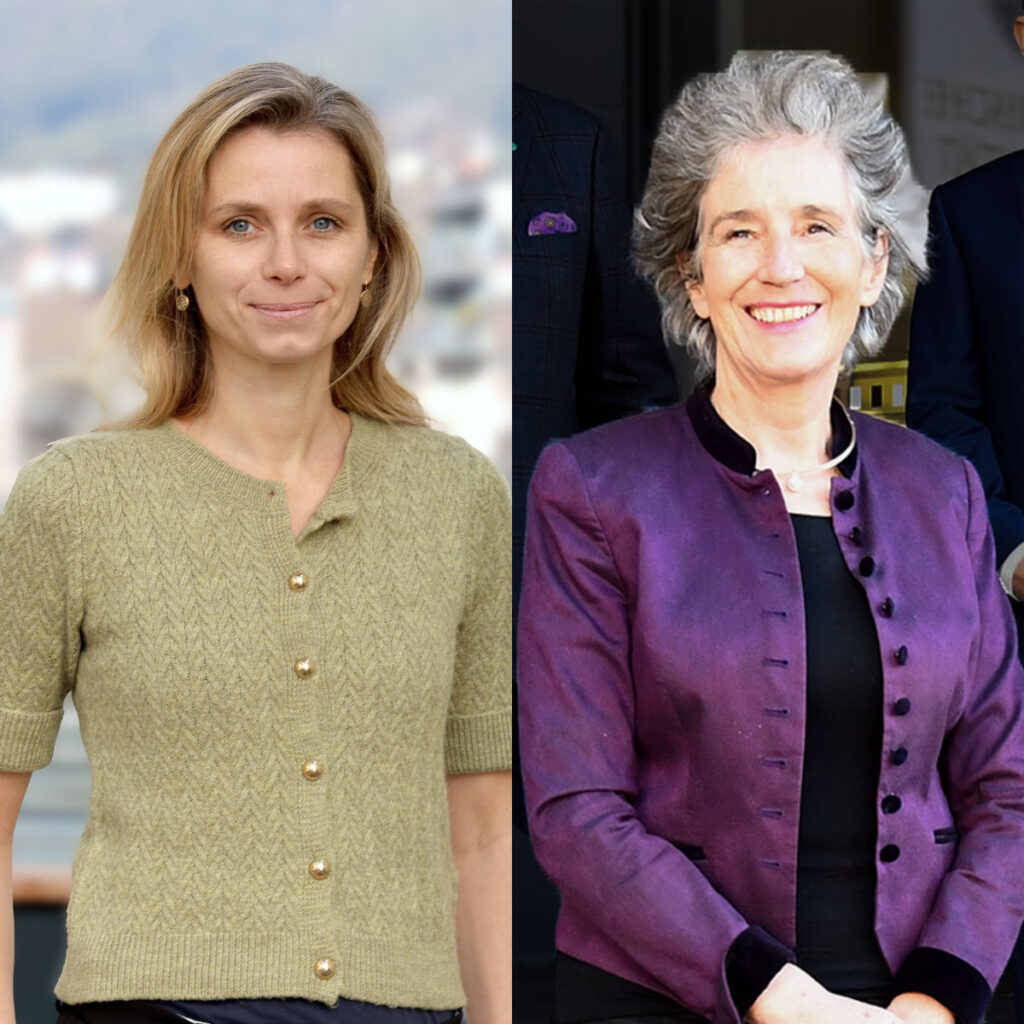
bis 2024: Univ.-Prof. Dr. Christine Bandtlow
Biocenter, Innrain 80-82
6020 Innsbruck
Email: Sabine.Liebscher@i-med.ac.at
Website: https://www.i-med.ac.at/neurobiochemistry/
Research Branch (ÖSTAT Classification)
106023, 106051, 106025, 301404, 301407
Keywords
circuit neuroscience, CNS disorders, decision-making, in vivo two-photon imaging, learning, neurodegeneration, neuronal networks, and prefrontal cortex
Research Focus
The institute’s neuroscience focus ranges from molecular and cellular studies to systems-level investigations, with a strong focus on translational approaches. We are interested in circuit mechanisms that govern complex physiological processes, such as decision making; in how circuits are affected in disorders of the brain to give rise to symptoms typical of a disease and to trigger and fuel degenerative processes; and in how to harness regenerative processes of the peripheral nerves.
General Facts
The Institute of Neurobiochemistry emphasizes an integrated, collaborative approach to neuroscience research. It combines molecular biology; cell biology; in vivo imaging and electrophysiology; and genetics to address complex questions in neurobiology. The Institute operates a state-of-the-art bioimaging core facility, providing access to advanced instruments and technologies for researchers at MUI and other institutions.
The institute consists of 3 labs.
- The Liebscher lab is addressing circuit mechanisms of CNS disorders, such as amyotrophic lateral sclerosis, spinocerebellar ataxia and autoimmune encephalitis
- The Passecker lab is investigating the neurobiological basis of decision-making processes and how it is altered in psychiatric diseases
- The Schweigreiter lab is studying axonal regeneration in the peripheral nervous system.
Research
Liebscher lab
Univ.-Prof. Sabine Liebscher, MD PhD
Shenyi Jiang, PhD student
Jackson Fontaine, PhD student
XiaoQian Ye, PhD student
Maja Überegger, lab manager
Dido Weber, TA
Sabine Liebscher’s research is focused on neuronal circuit dysfunction in CNS disorders, particularly neurodegenerative and autoimmune conditions. Her work combines advanced imaging techniques with molecular biology to identify therapeutic targets.
Research Focus Areas
1. Amyotrophic Lateral Sclerosis (ALS)
The Liebscher lab is investigating cortical hyperexcitability as an early driver of motor neuron degeneration. They are considering:
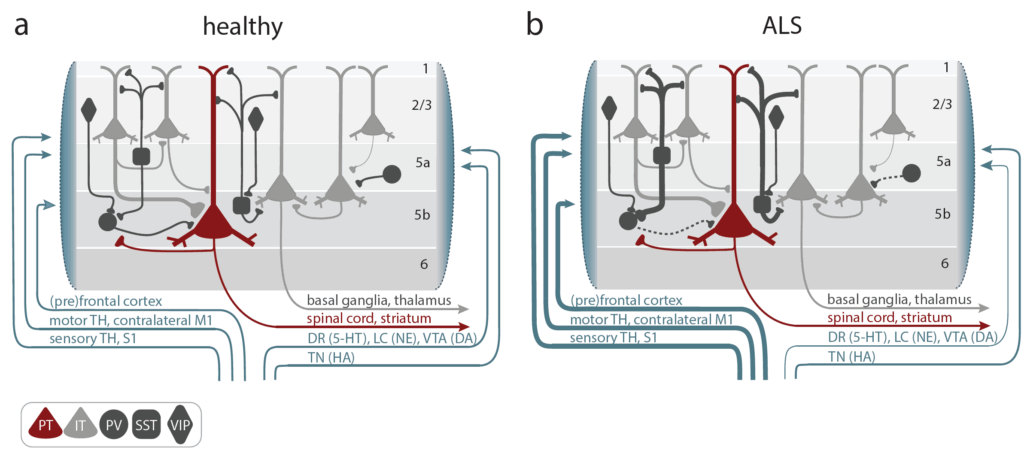
- Noradrenaline deficiency, which contribute to cortical hyperexcitability, potentially explaining excitotoxicity in ALS
- FUS protein accumulation, which triggers cortical neuronal hyperactivity and inhibitory synaptic defects
- Motor cortex microcircuit dysfunction, which we are studying by chronic in vivo two-photon calcium imaging in transgenic mouse models
- Molecular pathways involved in selective neuronal vulnerability to hyperexcitability
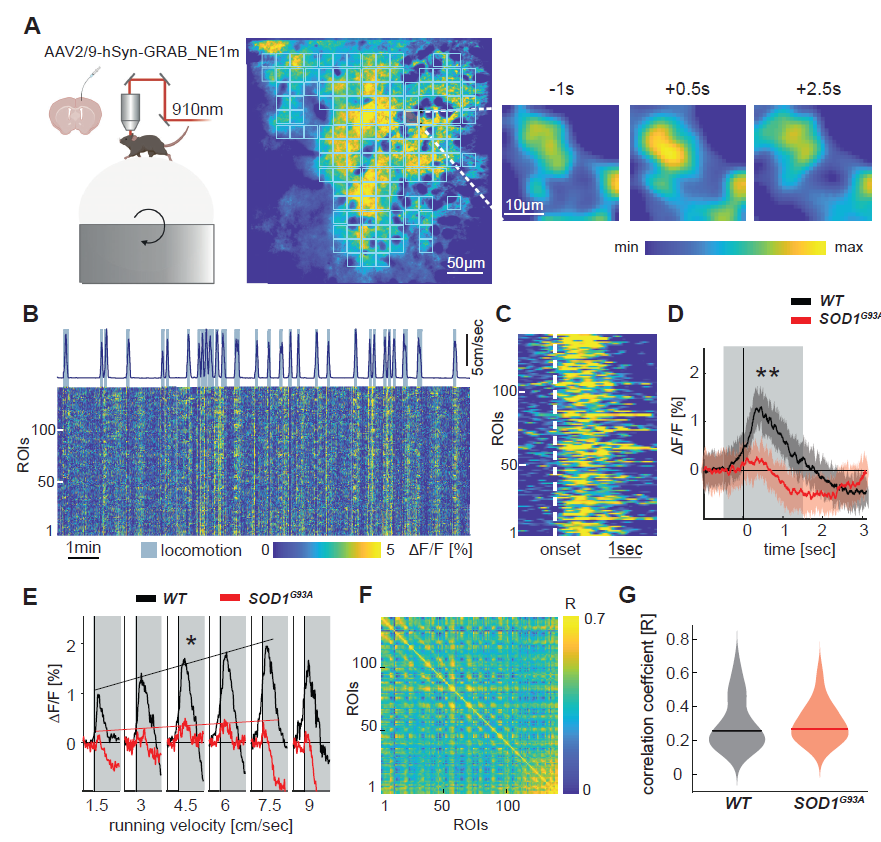
- Spinocerebellar ataxia (SCA)
- The role and cause of hyperactive molecular layer interneurons in Purkinje neuron dysfunction and degeneration in SCA1
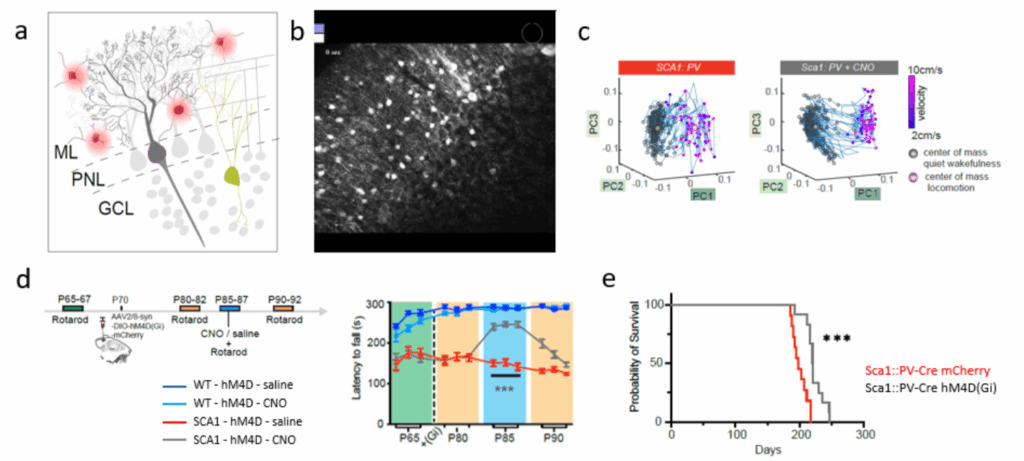
- Autoimmune Encephalitis
Her team is:
- using passive antibody transfer models to study virtual reality-based behavioural paradigms paired with calcium imaging to map neural network dysfunction that causes cognitive/psychiatric symptoms
- undertaking high-resolution in vivo imaging to study the impact of autoantibodies on synaptic structure and dynamics
- performing molecular investigations to identify cell type specific alterations underlying network dysfunction
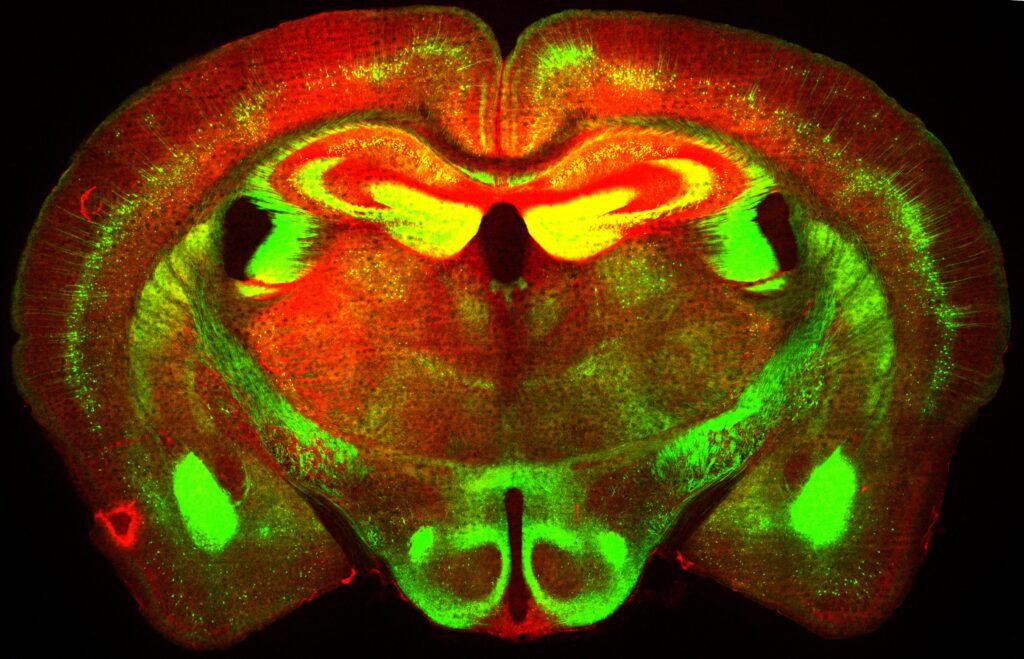
Passecker lab
Assist. Prof. Johannes Passecker, PhD
Aron Koszeghy, PhD, Post-Doc
Sofia Castro e Almeida, PhD student
Alexander Wallerus, PhD student
Nienke Blom, Project Manager
Judith Kathrein, Lead Project Manager
Franziska Vogel, Project Manager
Arsenii Petryk, Msc. student
Lukas Leitner, Diploma student
Leticia Wopfner, Diploma student
Ivan Vecchio, Diploma student
Anna Ruepp, Diploma student
Our research is focused on producing insights into how neuronal networks support cognitive control in both health and disease. How do we make decisions and how do the outcome and interpretation thereof affect our future goals, contextual interpretations and decisions? Our aims are to improve the neurobiological understanding of these processes and to develop better model systems for the stringent and efficient testing of potential rescue options of psychiatric disorders.
With colleagues and collaborators, we are pursuing an integrated approach, applying novel and state-of-the-art techniques to address the scientific questions behind the psychiatric maladaptation of our brains. Our techniques involve head-fixed and freely moving in vivo electrophysiology and behaviour, optogenetics, genetic model lines, RNA analysis, immunohistochemistry and behavioural testing combined with advanced statistical modelling, machine learning and deep-learning assisted analysis.
Our work includes an FWF funded project on how the prefrontal cortex communicates with the striatum on a function level for value-guided decision-making. We are developing new translational tasks and methods that utilize state-of-the art concepts, models and ML principles to provide new impulses for translational psychiatry research. We are part of the recently funded Austrian Cluster of Excellence, in which we and partners are exploring GABAergic circuits in higher cognition. Finally, we are participating in the Ebrains 2.0 project, in which we are coordinating and furthering education and training across the EBRAINS network, leveraging the advanced resources and expertise available. We are also managing the project’s calls, through which new data and workflows from the scientific community will be integrated into EBRAINS
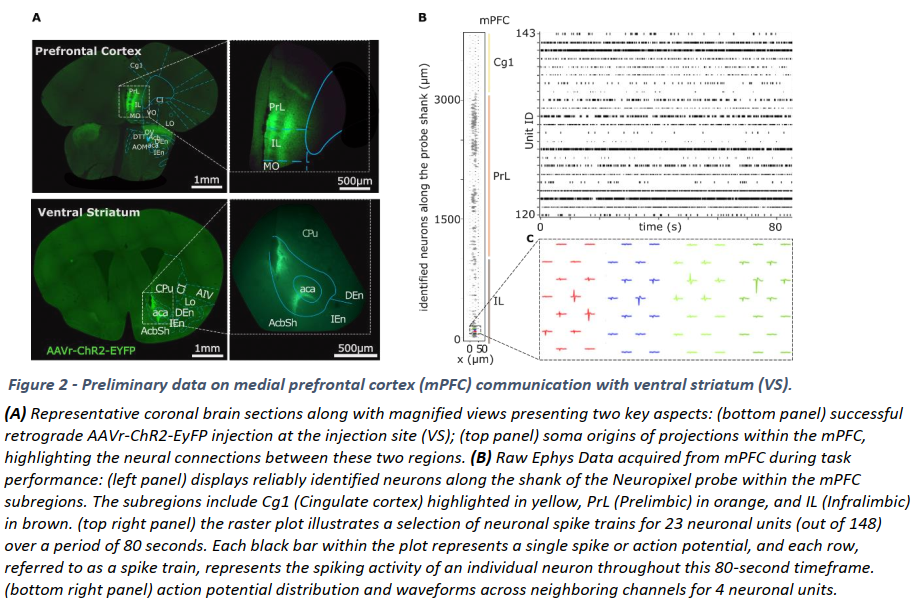
Schweigreiter lab
Assoc. Prof. Rüdiger Schweigreiter, PhD
Agata Lena Pruska, technician
Our primary research focus is on axonal regeneration in the peripheral nervous system (PNS). As traumatic nerve injuries involving nerve transection do not regenerate spontaneously, they require surgical intervention. Even with surgery, the outcomes are often poor and result in lifelong sensory and motor disabilities. The gold standard for surgical nerve repair is autologous nerve transplantation, typically using a segment of the sural nerve from the lower leg to bridge the lesion site and connect the proximal and distal nerve stumps. Axonal regeneration occurs naturally through the nerve graft without further intervention. However, autologous nerve grafts have several limitations, including donor site morbidity and limited availability. Despite technological advances, basic research to improve functional outcomes following traumatic PNI is lagging behind. We are developing an experimental peripheral nerve injury (PNI) model that closely mimics clinical conditions and enables clinically relevant conclusions. Its key features include:
- Transection-based lesion: the mouse sciatic nerve is transected and the nerve stumps fixed to a chitosan conduit. The procedure involves both interventionist and restorative surgery. While technically more demanding than rat surgery, work with mice offers access to numerous genetically modified reporter strains. Chitosan conduits are increasingly used in clinical nerve repair due to their excellent biocompatibility.
- Application of molecular compounds: soluble compounds can be applied directly at the lesion site before closing the conduit to promote axonal regeneration and sensory-motor recovery.
- Single axon visualization and quantification: we have developed a method to visualize and quantify regenerative nerve fibres within the conduit at the single axon level, providing high-resolution morphological data.
- Functional recovery assessment: animals undergo a battery of sensory-motor tests, including ladder walk, Von Frey and CatWalk analysis, to evaluate the functional improvement.
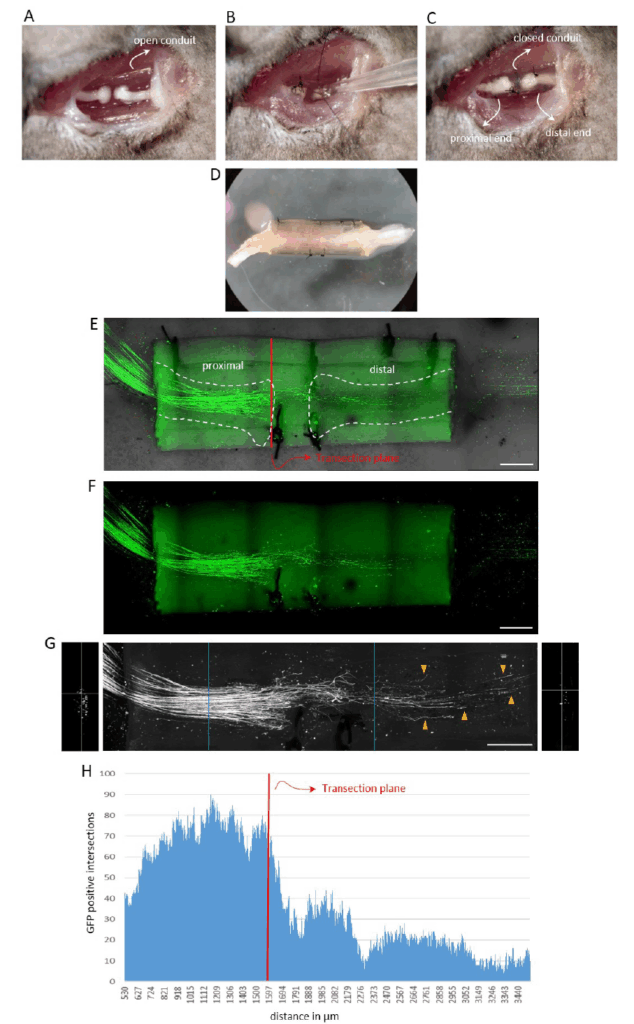
Copyright Rüdiger Schweigreiter
This nerve lesion model has direct clinical translatability and enables us to test various soluble compounds known to enhance axonal growth in vitro. Patients with severe limb injuries frequently experience lifelong sensory and motor deficits due to limited nerve regeneration. We are addressing this urgent clinical need by improving the functional restoration after traumatic PNI.
Pictures
Selected Publications
- Tzanoulinou S., Passecker, Stamatakis A., Diamantopoulou A., Translational behavioral approaches in animal models of psychiatry. Frontiers in Behavioral Neuroscience 2023, DOI: https://doi.org/10.3389/fnbeh.2023.1200691
- Geminiani, A., Kathrein, J., Yegenoglu, A. et al. , Interdisciplinary and Collaborative Training in Neuroscience: Insights from the Human Brain Project Education Programme. Neuroinform 22, 657–678 (2024). https://doi.org/10.1007/s12021-024-09682-6
- Scekic-Zahirovic, J., Benetton C., Brunet, A., Ye, X., Logunov, E., Douchamps, V., Megat, S., Andry, V., Kan, W.Y.V., Stuart-Lopez, G., Gilet, J., Trombini, M., Dieterle S., Sinninger, J., Fischer, M., Rene, F., Gunes, Z., Kessler, P., Dupuis, L., Pradat, P.F., Goumon, Y., Goutagny, R., Marchand-Pauvert, V. #, Liebscher S. # and Rouaux, C.#. (2024). Cortical hyperexcitability in mouse models and patients with amyotrophic lateral sclerosis is linked to noradrenaline deficiency. Science Translational Medicine, 16(738):eadg3665.
- Douthwaite C., Tietje C., Ye X., Liebscher S.. Probing cerebellar circuit dysfunction in rodent models of spinocerebellar ataxia by means of in vivo two-photon calcium imaging (2024). STAR protocols. 5(1):102911
- Kolabas Z.I., Kuemmerle L.B., …, Liebscher S., Hauser A.E., Gokce O., Lickert H., Steinke H., Benakis C., Braun C., Martinez-Jimenez C.P., Buerger K., Albert N.L., Hoglinger G., Levin J., Haass C., Kopczak A., Dichgans M., Havla J., Kumpfel T., Kerschensteiner M., Schifferer M., Simons M., Liesz A., Krahmer N., Bayraktar O.A., Franzmeier N., Plesnila N., Erener S., Puelles V.G., Delbridge C., Bhatia H.S., Hellal F., Elsner M., Bechmann I., Ondruschka B., Brendel M., Theis F.J., Erturk A..(2023). Distinct molecular profiles of skull bone marrow in health and neurological disorders. ;186(17):3706-25 e29
- Ceanga M., Rahmati V., Haselmann H., Schmidl L., Hunter D., Brauer A.K., Liebscher S., Kreye J., Pruss H., Groc L., Hallermann S., Dalmau J., Ori A., Heckmann M., Geis C. (2023). Human NMDAR autoantibodies disrupt excitatory-inhibitory balance, leading to hippocampal network hypersynchrony. Cell Rep.;42(10):113166
- Pilotto, F., Douthwaite, C., Diab, R., Ye, X., Al Quassab, Z., Tietje, C., Odriozola, A., Thapa, A., Buijsen, R., Lagache, S., Uldry, A.C., Heller, M., Muller, S., van Roon-Mom, W.M.C., Zuber, B., Liebscher S. # and Saxena, S#. (2023). Early molecular layer interneuron hyperactivity triggers Purkinje neuron degeneration in SCA1. Neuron
- Zambusi, A., Novoselc, K.T., Hutten, S., Kalpazidou, C.K., Schieweck, R., Aschenbroich, S., Silva, L., …, Liebscher, S., Schlegel, S., Aliee, H., Theis, F., Meiners, S., Kiebler, M., Dormann, D., Ninkovic, J., (2022). TDP-43 condensates and lipid droplets regulate the reactivity of microglia and regeneration after traumatic brain injury. Nature Neuroscience
- Schneider, J., Weigel, J., Wittmann, M.T., Svehla, P., Ehrt, S., Zheng, F., Elmazahi, T., Karpf, J., Basak, O., Ekici A., Reis, A., Knobloch, M., Alzheimer, C., Ortego de la O, F., Liebscher S. and Beckervordersandforth, R. (2022). Astrogenesis in the murine dentate gyrus is a life-long and plastic process mediated by proliferation of neural stem cells and local astrocytes. EMBO
- Empl L., Chovsepian A., Chahin M., Kan V., Salaam S.A., Marcantoni M., Ghanem A., Conzelmann K.K., Cai R., Ertürk A., Kreutzfeld M., Merkler D., Liebscher S. and Bareyre F. (2022). Adaptive plasticity of callosal neurons in the adult contralesional cortex following traumatic brain injury. Nature Communications
- D’Errico P., Ziegler-Waldkrich S., Aires-Mofreita V., Hoffmann P., Mezö C., Erny D., Liebscher S., Kierdorf K., Straszewski O., Prinz M. and Meyer-Luehmann M. (2022). Microglia contribute to the propagation of Aβ pathology into unaffeced brain tissue. Nature Neuroscience
- Gunes ZI., Kan VWY., Jiang S., Logunov E., Ye X. and Liebscher S. (2022). Cortical Hyperexcitability in the Driver’s Seat in ALS. Clinical and Translational Neuroscience
- Steffens H., Mott A. C., Li S., Wegner W., Svehla P., Kan W.Y.V., Wolf F., Liebscher S.# and Willig K.# (2021). Stable but not rigid: Chronic in vivo STED nanoscopy reveals extensive remodeling of spines, indicating multiple drivers of plasticity. Science Advances # equal contribution
Scekic-Zahirovic J., Sanjuan-Ruiz I., Kan V, Megat S., De Rossi P., Dieterlé S., Cassel R., Kessler P., Wiesner D., Tzeplaeff L., Demais V., Muller H.P., Picchiarelli G., Mishra N., Grosch S., Kassubek J., Rasche V., Ludolph A., Boutillier A.-L., Roselli F., Polymenidou M., Lagier-Tourenne C., Liebscher S.# and Dupuis L.# (2021). Cytoplasmic accumulation of FUS triggers early behavioral alterations linked to cortical neuronal hyperactivity and inhibitory synaptic defects. Nature communications # equal contribution
Selection of Funding
- 2024-2029: Cluster of Excellence 10.55776/COE16 – Grant, Title: Neuronal Circuits in Health and Disease; Role: Johannes Passecker Task Leader, PI, Funding Agency: Austrian Science Fund (FWF), AT
- 2024-2026: P 101147319 Title: Ebrains2.0, Role: Johannes Passecker: Task Leader, Budget: Funding Agency: European Union, EU
- FWF Project # P33411-B
- 2024- 2026: Tambourine-Milken ALS Breakthrough Research Fund
- 2023 – 2025: Target ALS foundation
- 2025 – 2026: Frick foundation
- 2023 – 2026: DFG SynAbs Forschergruppe
- 2022 – 2025: ANR- DFG grant ‘FrequALS’ (German coordinator)
- 2023 – 2025: DFG individual grant
Collaborations
- Dr. Joseph Gogos, Zuckerman Institute, Columbia University, New York, USA
- Dr. Joshua Gordon, Chair of the Department of Psychiatry, Columbia University Vagelos College of Physicians and Surgeons, Executive Director of the New York State Psychiatric Institute, New York, USA
- Dr. David Kupferschmidt, National Institute of Neurological Disease & Stroke, Bethesda, USA
- Dr. Nace Mikus, University of Aarhus, Aarhus, DK
Prof. Claus Lamm, University of Vienna
- Prof. Caroline Rouaux & Prof. Luc Dupuis, INSERM, Strasbourg, France
- Prof. Smita Saxena, NextGen Precision, University of Missouri School of Medicine, Columbia, Missouri, USA
- Prof. Brian McCabe & Dr. Bernard Schneider, EPFL, Lausanne/Geneva, Switzerland
- Prof. Romana Höftberger, Medizinische Universität Wien, Vienna, Austria
- Sebastian Munck, KU Leuven, Belgium