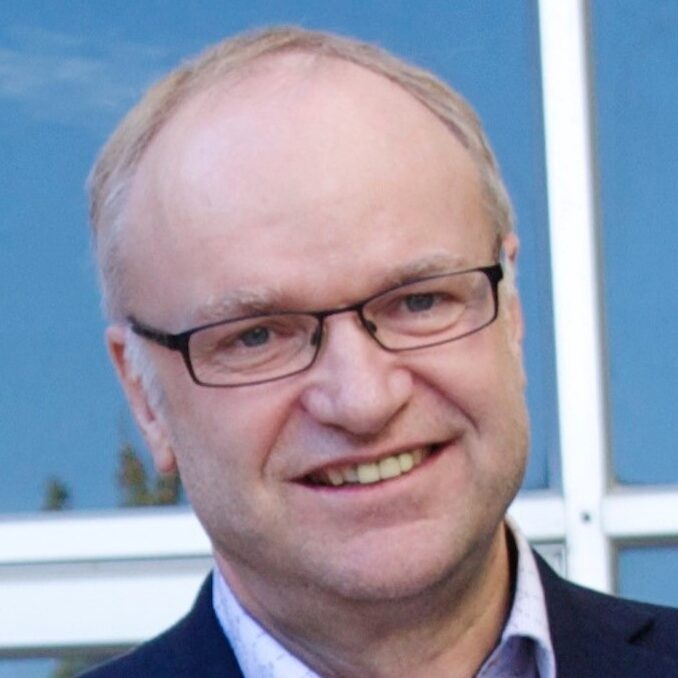
Innrain 80-82
6020 Innsbruck
Fax: +43 512 9003 73100
Email: ludger.hengst@i-med.ac.at
Website: https://www.i-med.ac.at/imcbc/medclinchemfolder/medclinchem.html
Research Branch (ÖSTAT Classification)
104002, 106002, 106037, 106052, 3013, 301303, 301904, 301211, 301114
Keywords
capillary electrophoresis, Cell Cycle Control, Cell Proliferation, Immuno-metabolism, Mass Spectrometry, Protein Kinases, Protein Microanalysis Facility, Toxicology, and Translational Control
Research Focus
General Facts
The institute hosts two research groups led by Ludger Hengst and Johanna Gostner and a protein core facility led by Bettina Sarg. Using a broad range of cell biological and molecular biological techniques, the cell cycle and cell proliferation group investigates molecular mechanisms that govern the decision between cell proliferation and cell cycle exit to quiescence or differentiation in normal mammalian cells and tissues and in cancer models. Here, we focus on the regulation of cyclin-dependent kinase (CDK) activities and CDK inhibitors and we study mechanisms of transcriptional, translational and post-translational control. The biochemical immuno-toxicology group focuses on cellular responses to chemical exposure and its immuno-metabolic consequences. The protein core facility develops and establishes novel analytical methods and technologies. This facility provides a wide platform of services such as HPLC, capillary electrophoresis and MS-based analysis and quantification of proteins from cells, plasma or tissues as well as elemental analysis using graphite furnace atomic absorption spectrometry (GF-AAS) to measure specific elements in tissue biopsies, blood or urine.
Research
Cell Cycle and Cell Proliferation
Ludger Hengst
Precisely coordinated cell division and differentiation processes are essential for the growth, development and integrity of multicellular organisms. Before cells commit to division or quiescence, they are exposed to a flood of diverse and sometimes conflicting signals that aim to regulate cell growth, differentiation, cell proliferation or cell fate. Multiple external as well as internal signals can impinge on the central cell cycle control machinery, in order to promote or block cell proliferation. These signals all need to be properly processed and integrated, in order to control cell proliferation and quiescence and to maintain body and organ homeostasis. Incorrect signal interpretation, processing or integration can lead to hypo- or hyper-proliferative disorders, including diseases such as cancer or anaemia.
The decision to continue proliferation or to exit from the cell cycle into quiescence is usually made during a specific window of the eukaryotic cell division cycle. This cell cycle can be subdivided into four phases: gap phases G1 and G2 separate DNA replication during the S-phase from segregation of the duplicated DNA and other cellular components in the M-phase (mitosis and cytokinesis). Cells can decide to withdraw from proliferation or commit to another round of cell division until they progress over the so-called restriction point, a specific point in phase G1 (Fig. 1). Progression over the restriction point renders cells mitogen-independent and they become committed to undergoing another complete cell cycle including S-phase and mitosis.
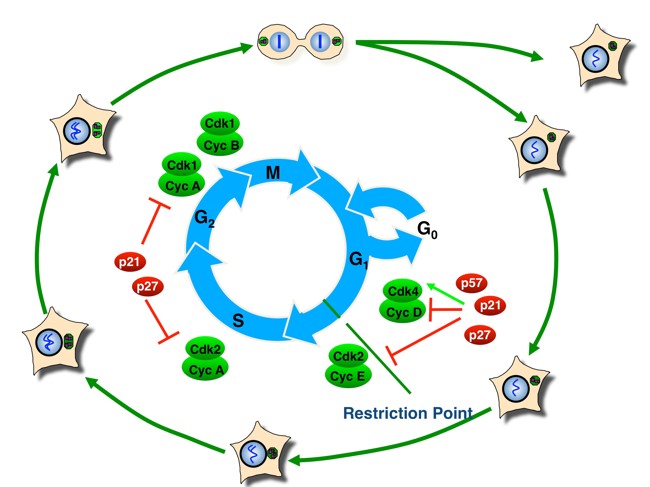
We investigate molecular mechanisms that link diverse signalling networks to the central cell cycle control machinery. At the core of this machinery is a conserved family of protein kinases called cyclin-dependent kinases (CDKs). CDKs are activated by the binding of a positive regulatory subunit, the cyclin. Sequential activation and inactivation of specific CDK complexes is required for cell cycle progression. p21 (CDK-interacting protein, Cip1), p27 (kinase-inhibitory protein, Kip1) and p57 (Kip2) constitute one of two families of CDK inhibitors (CKI) that bind to CDKs and control CDK kinase activity. Their expression, localisation and modification play a central role in regulating CDK kinase activity, especially during phase G1 and the choice between proliferation and cell cycle exit. In addition to their canonical function in CDK kinase regulation, these inhibitors can also exert CDK-independent and cell cycle-independent functions. For example, CDK inhibitor p27 can regulate cell motility and cell migration, which links this tumour suppressor protein not only to cell proliferation but also to cancer metastasis. Interestingly, some non-canonical functions of Cip/Kip proteins, such as transcriptional control, can be both CDK-dependent and CDK-independent.
Among others, we identified CDK inhibitor protein p27Kip1. Its activity, localisation or stability can be regulated by diverse mitogen signalling pathways. We investigate how these pathways control Cip/Kip family protein expression, localisation, modification, activity or function and we study their physiological roles in normal cells and cancer cells.
p27 regulates cell cycle progression over the restriction point. Abundant p27 binds and inactivates CDKs and can prevent cell proliferation. The CDK inhibitor protein becomes unstable upon cyclin/CDK2 activation as cells traverse the restriction point and progress towards the S-phase. A positive feedback loop couples p27 ubiquitin-dependent degradation to CDK activation (Fig. 2). The molecular mechanism that can initiate this feedback loop in the presence of abundant CDK inhibitors and thus inactive CDKs has long remained an enigma.
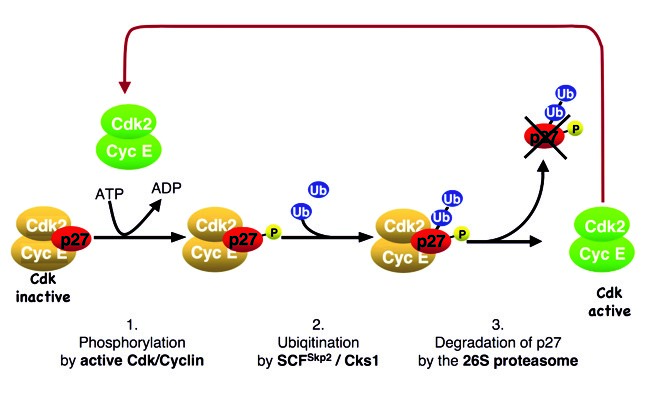
We observed that oncogenic tyrosine kinases, including BCR-Abl, Src and JAK2, can activate p27-bound CDKs by phosphorylating the inhibitor. Upon phosphorylation, an inhibitory helix of p27 is ejected from the catalytic cleft of the CDK and allows the p27-bound CDK complex to bind ATP and to phosphorylate substrates. One of these substrates is p27 itself. Phosphorylation of p27 by the bound CDK generates a phosphodegron, which can initiate SCF-Skp2-mediated ubiquitin-proteasomal degradation of the CDK inhibitor (Fig. 3). Using this mechanism, which can be abused by oncogenic kinases, mitogen signals can inactivate and destabilise inhibitor p27 and thereby promote CDK activation and cell cycle progression.
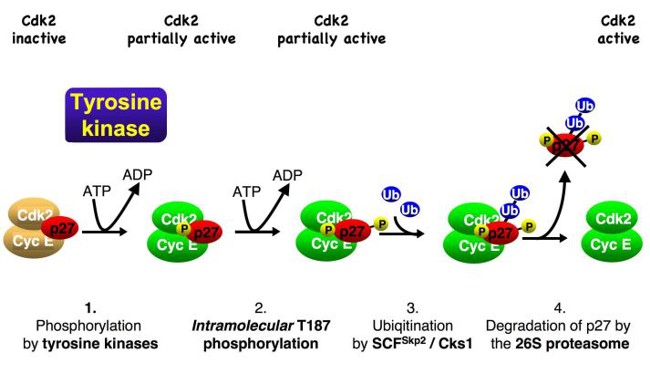
Ongoing research
Current research is focusing on the regulation of cell cycle progression through phase G1. We investigate mechanisms of transcriptional control by Cip/Kip proteins, translational control, temporal and spatial regulation of CDK-inhibitory proteins and the control of ubiquitin E3 ligase complexes, such as SCF-Skp2, the molecular mechanism of statin-induced cell-cycle arrest and cell-cycle control by erythropoietin (Epo) and its receptor EpoR. In addition, we investigate the physiological role of highly conserved small peptides encoded in uORF sequences of mRNAs encoding cell cycle regulatory proteins.
Major achievements
We have discovered a novel mechanism that controls p27 stability by means of proteasomal degradation and identified novel pathways that control the subcellular localisation of p27. We have elucidated the molecular mechanisms that induce p27 stabilisation and Skp2 degradation in the presence of statins. We have also identified novel p27 mRNAbinding proteins, which are components of stress granules and which regulate the IRES and cap-dependent translation of p27. We have further investigated transcriptional functions of Cip/Kip protein p57Kip2, which can co-activate c-Jun/AP-1 or FHL2-mediated transcription.
Current research projects
- Function and regulation of CDK-inhibitory proteins.
- Role of translational control in the choice between cell proliferation and withdrawal from the cell cycle.
- Regulation of cytokine receptor signalling.
- Ubiquitin E3 ligase regulation in response to stress.
- Transcriptional functions of Cip/Kip proteins.
- Function of small peptides encoded in the uORFs of mRNAs encoding cell cycle-regulatory proteins.
Biochemical Immuno-toxicology
Johanna Gostner
Understanding of biochemical and molecular processes is a prerequisite to deciphering responses to a changing environment. Increased exposure to environmental chemicals has an impact on human health. Nutrition and lifestyle factors may further modify susceptibilities.
Ongoing research
By integrating analytical and bio-chemistry, cell biology and immunological approaches together with biotechnological tools, we aim to investigate the cellular response to chemicals, e.g. volatile compounds that affect air quality, compounds applied in everyday or care products, or phytochemicals, in order to gain a better understanding of the complex processes involved in sensitisation, irritation and adaptation. We aim to validate in vitro methods, to establish workflows for in vitro to in vivo extrapolation of effects and to evaluate biomarkers in occupational settings. Moreover, we have a keen interest in immuno-metabolism, primarily focusing on amino acid and pteridine biochemistry – pathways that have been shown to be susceptible to modulation, e.g. by drugs, chemicals, natural products, food or exercise. Several metabolites of the aforementioned pathways serve as sensitive and reliable biomarkers of disorders associated with chronic inflammation and provide a link between immuno-toxicity, immuno-deficiency and neuro-psychiatric changes.
Pictures
Selected Publications
The Hengst Laboratory: Cell Cycle and Cell Proliferation Control
- Inability to phosphorylate Y88 of p27Kip1 enforces reduced p27 protein levels and accelerates leukemia progression. Jäkel Heidelinde, Taschler Martin, Jung Karin, Weinl Christina, Pegka Fraga, Kullmann Michael Keith, Podmirseg Silvoio Roland, Dutta Sayantanee, Moser Markus, Hengst Ludger. Leukemia. 2022; 36(7):1916-1925. PMID: 35597806 doi: 10.1038/s41375-022-01598-x.
- The CDK inhibitor p57Kip2 enhances the activity of the transcriptional coactivator FHL2. Kullmann, Michael K.; Podmirseg, Silvio R.; Roilo, Martina; Hengst, Ludger, SCIENTIFIC REPORTS: 2020; April 28; 10 (1): 7140. doi:1038/s41598-020-62641-4.
- Stimulation of c-Jun/AP-1 activity by the cell cycle inhibitor p57Kip2. Kullmann, Michael K.; Pegka, Fragka; Ploner, Christian; Hengst, Ludger. Frontiers in Cell and Developmental Biology: 2021; March doi:10.3389/fcell.2021.664609.
- Cold-inducible RNA-binding protein (CIRP) induces translation of the cell-cycle inhibitor p27Kip1. Roilo, Martina, Kullmann, Michael K., Hengst, Ludger. NUCLEIC ACIDS RESEARCH (2018). 46(6): 3192-3210.
The Gostner Laboratory: Biochemical Immunotoxicology
- A Chlorophyll-Derived Phylloxanthobilin Is a Potent Antioxidant That Modulates Immunometabolism in Human PBMC. Karg CA, Parráková L, Fuchs D, Schennach H, Kräutler B, Moser S, Gostner JM. Antioxidants (Basel). 2022 Oct 19;11(10):2056. doi: 10.3390/antiox11102056. PMID: 36290779
- Contradictory effects of chemical filters in UV/ROS-stressed human keratinocyte and fibroblast cells. Hofer, Stefanie; Stonig, Marlies; Wally, Verena; Hartmann, Anja; Fuchs, Dietmar; Hermann, Martin; Paparella, Martin; Ganzera, Markus; Gostner, Johanna M.: ALTEX-ALTERNATIVES TO ANIMAL EXPERIMENTATION. 2019; 36(2); 231-244. PubMed: 30488083 doi: 10.14573/altex.1808201
- Dietary digestible carbohydrates are associated with higher prevalence of asthma in humans and with aggravated lung allergic inflammation in mice. Musiol S, Harris CP, Karlina R, Gostner JM, Rathkolb B, Schnautz B, Schneider E, Mair L, Vergara EE, Flexeder C, Koletzko S, Bauer CP, Schikowski T, Berdel D, von Berg A, Herberth G, Rozman J, Hrabe de Angelis M, Standl M, Schmidt-Weber CB, Ussar S, Alessandrini F. Allergy. 2023 May;78(5):1218-1233. doi: 10.1111/all.15589. PMID: 36424672
Selection of Funding
- FWF WEAVE, I 6122: UVISION 1.1
- FWF Doc 82/10 :Cellular Basis of Diseases: Molecular Control of Metabolism and Inflammation
- FWF TAI 432 1000 Ideen Programm: Neue Aufgaben für kleine uORF-kodierte Peptide.
- FER: Formaldehyde exposure and cellular regeneration. Cooperation with Egger
- Coupling capillary electrophoresis to mass spectrometry for protein and proteome analysis. Industrial Project with AB SCIEX; U.S.A.
- Investigation of in-vivo O-Glycosylation of the low abundance marker NT-proBNP by Affinity Proteomics Methods and Mass Spectrometry in blood plasma from patients with severe heart failure. Industrial Project with Roche Diagnostics GmbH Penzberg, Germany
Collaborations
- Joyce M Slingerland, University of Miami, USA
- Richard W. Kriwacki, St. Jude Hosp. of Sick Children, Memphis, USA
- Pierre Roger, Universite Libre de Bruxelles, Bruxelles, Belgium
- Stephen J. Elledge, Harvard University, Boston, USA
- Joan Conaway, Stowers Institute Kansas City, USA
- Drorit Neumann, Tel Aviv University, Israel
- Terrance Lappin, Queens University Belfast, UK